A synchrotron facility is a laboratory where scientists can examine molecular structures and surfaces in a far more detailed way than before. Researchers in areas such as biology, physics, chemistry, environment, geology, engineering and medicine can utilise this technology.
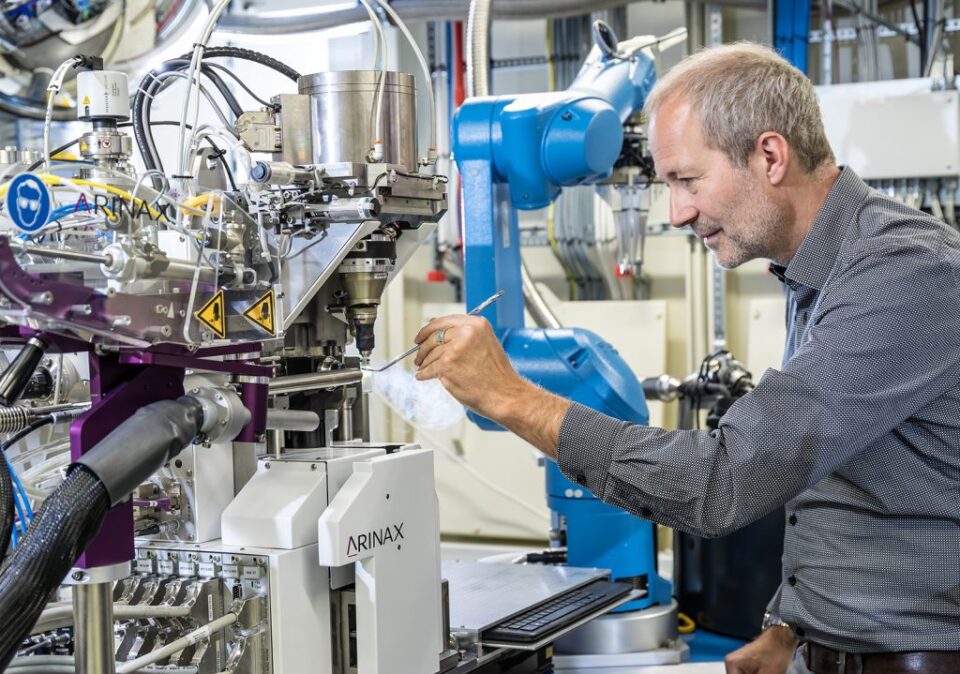
A synchrotron is an extremely powerful source of X-rays. It builds on the physical phenomenon that a moving electron emits energy when it changes direction. If the electron is moving fast enough, the emitted energy, called synchrotron radiation, is at X-ray wavelength. Synchrotron radiation is millions of times more brilliant than anything a physician’s X-ray machine can produce. The technology provides opportunities to make new discoveries and products in fields such as materials, medicine and the environment.
Various techniques are used in the experiments: imaging, spectroscopy and scattering. The techniques are often combined and together they offer researchers opportunities to study and develop new drugs, efficient batteries and solar cells, as well as alloys, paper, fabrics and plastics with new functions. Pollutants in water and soil can be measured in order to identify new ways to tackle contamination. Historical and archaeological objects can be examined without damaging them. Healthy and diseased cells and tissue can be analysed as a basis for developing new treatments.
However, the majority of the experiments are for basic research, which aims to give us insights into the workings of the smallest components in materials. This knowledge is necessary for pursuing more applied research such as the examples mentioned above.
Imaging provides knowledge on what materials look like from the outside, as in a photograph, or from within, as in an X-ray image. The method delivers an image or film in two or more dimensions. The techniques used include microscopy and tomography. With nanometre resolution, it is possible to see an electronic component that has been built using nanotechnology. The technique can be used to see how the nanostructures within the component are affected when it is used, providing insights into how better and more efficient components can be built, such as more reliable catalysts.
Spectroscopy provides knowledge of chemistry and where the components in matter are positioned in relation to each other. Spectroscopy involves methods based on measuring the response that arises in the material when it is illuminated with various types of light. The techniques used include photoelectron spectroscopy and fluorescence. These techniques can be utilised to identify the chemical composition of an examined sample. Spectroscopy can be used to identify which trace substances are present in plant samples. This can, for example, provide a better understanding of how metals are taken up by plants and the resulting environmental impact. It is also possible to see how atoms and molecules interact on a catalytically active surface in order to develop more effective catalytic converters in exhaust emission control systems.
Scattering provides knowledge on the structure of atoms or molecules. Scattering is a term that covers various phenomena that arise when light meets a material. The light can be reflected or change direction when it passes through the material. These changes can be measured. The techniques used for scattering include X-ray crystallography and powder diffraction. These techniques can be used to identify how the atoms or molecules are positioned in relation to each other, which is important for the characteristics – mechanical, magnetic, electronic, etc. – of the matter or material. Scattering can be used to observe battery materials in order to see how the atoms move when the battery is charged and thereby gain a better understanding of how improved, lighter and cheaper batteries can be designed. It is also possible to see how the structure of a material changes when it is subjected to mechanical forces. This provides knowledge that can be used to develop new, stronger materials with higher breaking strength.
Synchrotron radiation is produced by electrons that circle inside an accelerator ring. When these electrons, which travel at nearly the speed of light, are guided into a curve by deflecting magnets, they emit a highly intense and collimated beam of light whose spectrum ranges from infrared light to X-rays.
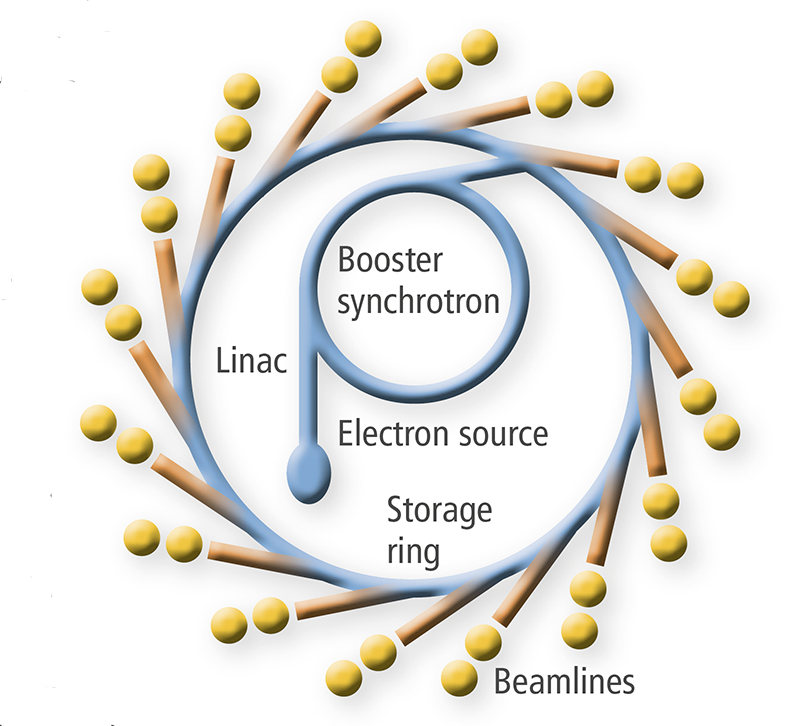
Even more effective than deflecting magnets are special magnets known as wigglers and undulators. They are used in storage rings and consist of a series of alternating pairs of magnets extending over several metres. This magnetic “racetrack” forces the superfast electrons to speed along a narrow zigzag channel. Due to a large number of successive magnetic poles, the electrons emit a much more intense beam of light than that produced with a single deflecting magnet. Use of a wiggler generates synchrotron radiation up to 100 times as intense as that produced with deflecting magnets. In a certain mode of operation, the intensity at specific wavelengths can even be 1000 times higher because the wave trains reinforce one another. As a result, the wiggler is transformed into an undulator.